01dragonslayer
Iron Killer
Mad Referrer
Jacked Immortal
EG Auction Sniper
VIP Member
Shout Master
Mutated
Fully Loaded
- EG Cash
- 1,113,703
Creatine has a very long and controversial history. Creatine ( methyl guandino-acetice acid) was discovered by French chemist Michel Eugene Chevreul in 1832. Chevreul was studying different metabolites found in meats when he first isolated creatine; he named it after the Greek word for flesh, Kreas. (16) Unknown to Chevereul at the time, creatine would become the most popular dietary supplement in the world. (1) Justus von Liebig confirmed Chevereul’s discovery in 1847 and conducted additional research on creatine and animal physiology. Liebig would make several interesting findings while conducting his research. He discovered that wild foxes contained ten times more creatine in their skeletal muscle than foxes in captivity did. He concluded that physical exercise caused an increase in creatine concentration in skeletal muscle. (1)
In 1927 phosphocreatine, a derivative of creatine, was discovered. This breakthrough discovery came from Fiske and Subbarow. (1) Their research established the fact that phosphocreatine concentrations in skeletal muscles decreased during contractions, and concentrations rose again during resting periods. (1) They also discovered the role phosphocreatine plays in energy production. After Fiske and Subbarow’s findings, little research was conducted on creatine until 1992 when Chanutin reported that upon creatine ingestion, total creatine concentrations in skeletal muscle rose. (1) This finding meant that supplemental creatine could be absorbed directly into skeletal muscle. This study has been confirmed by other studies including the one conducted by Harris et al in 1992, which is considered the major persuading study that began the revolution of creatine supplementation. (17) As a result of the Harris et al study, the amount of research being conducted on creatine increased rapidly. The purpose of this paper is to demonstrate how creatine can be used as a sports supplement using a chemical standpoint, and to investigate differing factors that contribute to the effectiveness of creatine supplementation.
In recent years, creatine has become an interesting topic outside of the performance enhancing field and into the medical field. Evidence has been revealed that suggests creatine may have importance in the treatment of several disorders such as Parkinson’s disease, neuromuscular diseases, brain diseases, muscular dystrophies, and even heart disease. (2,19) This evidence has broadened the possibility of creatine outside of improving athletic performance and into saving lives.
It is important to note that when ATP is being dephosphorylated into ADP, resynthesis of ATP is being achieved at the same time inside of the muscle. This is where creatine becomes a crucial factor in the energy production of muscle cells in the body. During contraction, this resynthesis of ATP from ADP is accomplished through the degradation of phosphocreatine. This reaction is presented in figure 1: (4)
The Creatine Kinase Reaction Presented in this image is the creatine kinase reaction which occurs in skeletal muscle to resynthesize ATP. As one can see phosphocreatine combined with ADP yields creatine (Cr) and ATP to be used for energy production. Adapted from Wyss and Kaddurah-Daouk (2000). (4)
As a result of this direct interaction between phosphocreatine and ADP, phosphocreatine concentrations is the limiting factor in the resynthesis of ATP. With this knowledge of how skeletal muscle uses the creatine kinase reaction to produce energy, the supplementation of creatine can now be investigated. Many factors regarding creatine supplementation will be explored below, as well as the effectiveness of the creatine supplementation on performance enhancement.
The first factor that is to be investigated is the contribution creatine, in its pure form, has on maintaining energy levels. Even though creatine does not directly react with ADP to produce ATP, there is a pathway by which creatine assists in the resynthesis of ATP as shown in figure 1. Creatine performs this task by acting as a buffer to the reaction that occurs during the resynthesis of ATP. (5) It has been proven that upon creatine supplementation, the total concentration of phosphocreatine in skeletal muscle increases. (6) Therefore, creatine supplementation does not allow the concentration of phopsocreatine to decrease low enough where the equilibrium will shift away from the production of ATP under Le Chatelier’s principal. This elevated phosphocreatine also shifts the equilibrium towards production of ATP under Le Chatelier’s principal as well. This buffering system, in turn, allows for much faster and more efficient production of ATP, causing the overall of availability of ATP to rise.
Although one may wish to consider creatine as the perfect supplement for energy production in muscle tissue, reduced energy production is not the only reason muscle fibers become fatigued. The other factor to consider, with regard to muscle fatigue, is the build up of lactic acid (2-hydroxypropanoic acid) during contraction. As the muscle contracts the demand for ATP begins to rise. As ATP use increases, lactic acid is produced in the muscle tissue at a rate faster than the muscle can remove it. This inability to remove lactic acid fast enough causes the concentration of lactic acid in the muscle tissue to rise. As the concentration rises, this lowers the pH of the muscle with has been linked to cause a fatigue effect in the muscle. (7) However, it is important to note that according to Katz et al the fatigue that is experienced during short-term contraction is more dependant on low phosphocreatine concentrations rather than the increased concentrations of lactic acid. (8) This result has been confirmed by Saugen et al. (18) These findings show the importance phosphocreatine plays in the amount of energy a muscle can produce during contraction.
Another aspect of creatine in the human body to consider is which type of muscle fiber consumes more creatine during contraction. According to Casey and Greenhaff, the two different types of muscle fibers found in skeletal muscle, type I and type II, consume creatine in different amounts. (3) Type I fibers are considered slow-twitch fibers and are more useful when conducting aerobic exercise such as long distance running or biking. Type II fibers are considered fast-twitch fibers and are used during short maximum effort muscle contraction such as lifting weights. They report that the use of phosphocreatine in type II fibers can be as much as 33% higher than the use in type I fibers. Since the major source of energy for type I fibers is triglycerides and ATP for type II fibers, it can be speculated that the supplementation of creatine would be more beneficial for short explosive anaerobic exercises, than it would be for longer aerobic exercises. (3)
It is also important to investigate the maximum amount of creatine, and ultimately phosphocreatine, which can be stored inside muscle fibers. The average person who consumes a normal diet consisting of protein, carbohydrates, and fats has a base creatine level of approximately 120mmol of creatine per kilogram of dry mass. (5) This amount can be substantially lower depending on the individual’s diet. For example, a vegetarian has lower creatine levels because of the absence of meat in the individuals diet. According to Paddon-Jones et al, the maximum concentration of creatine in skeletal muscle that can be achieved through supplementation is 160mmol per kilogram of dry mass. (5) This concentration cannot be increased by additional creatine supplementation. Once this concentration has been reached, additional ingested creatine will be excreted.
In addition to the proposed energetic effects creatine has on skeletal muscle, it has been found to play a role in the mechanisms that control muscle protein synthesis. In one study, researchers discovered that elevated creatine concentration of skeletal muscle cells leads to an increase in myosin heavy chain synthesis. (9) These studies were conducted in vitro using tissue cultures. The results of Ingwall’s et al study are presented in Table 1.
Total Percentage of Myosin Heavy Chain Synthesis after Creatine Supplementation in Tissue Culture Cells. Culture cells were incubated for a four hour period where they were injected with a 5mM concentrated solution of creatine and warm water. As one can see the synthesis of myosin heavy chain raised drastically when the creatine was introduced into the tissue cultures. The total protein synthesis and total myosin heavy chain synthesis were measured using 3H decay analysis. (9)
As one can see, the increase of creatine concentrations raised the amount of myosin heavy chain and the total protein synthesized inside of the cell. These findings were later confirmed by many similar studies conducted by Ingwall et al. (19) Because myosin heavy chain is the major protein that initiates muscle contraction, these results give evidence that creatine promotes maximum muscle contraction through two different mechanisms: an energetic effect as well as promoting the synthesis of myosin heavy chain. Conversely, it is also important to note that some studies have not been able to find that creatine supplementation improves overall protein synthesis. However, one such study was able to find that creatine supplementation possibly decreased the rate of protein catabolism, or the rate in which protein is broken down inside of the body. (19)
Even though the theoretical possibilities of creatine seem to be amazing, the question still remains as to whether creatine supplementation can improve athletic performance. There have been countless studies conducted on creatine supplementation, and surprisingly not, they have revealed conflicting results. Casey and Greenhaff found that creatine supplementation of 20g per day for five days resulted in improved performance in maximum isokinetic leg extensions of up to 4%. (3) Casey et al discovered that with the same supplementation regimen as Casey and Greenhaff, nine male subjects increased their total work production during several maximum isokinetic exercises by an average of 4%. (10) Harris et al conducted tests on mid-range trained runners at Tartu University, where they found creatine supplementation of 20-30g per day for five days significantly improved the runners’ times in their respective race compared to a placebo group. (11) This study conducted by Harris et al indicated that creatine was not only useful in short term maximum muscle contraction, but also useful in exercises that use more type I muscle fibers such as mid-ranged races.
Although there have been a large number of studies conducted where creatine supplementation was shown to improve athletic performance, there were many other studies where no such evidence was found. One such study was conducted by Syrotuik et al, in which they investigated the performance of rowers. (12) The study found that through an eight week period, there was no significant increase between the subjects supplementing with creatine and the placebo group. Similar results were obtained by Odland et al during 30 seconds of maximum exercise. (13) Because many of these studies tend to contradict each other, it is important to note some of the aspects of the studies presented above. The study conducted by Odland et al only lasted for three days, in which many feel is too short for the phosphocreatine concentrations inside the muscle to be properly elevated. The normal time period allowed for creatine concentration in the muscle to reach a maximum level and stay constant is five days of supplementation. This shortened time period could possibly lead to inaccurate results, and incorrect conclusions. The study performed by Syrotuik et al lasted significantly longer than the previous study. However, this study used the same training volume for both the supplemented group and the placebo group. As the supplemented group phosphocreatine concentrations began to rise, they were unable to increase their training volume if they wished throughout the study. Because the training volume was held constant for both groups in the study, this could have led to inaccurate results.
Through the results discussed above, it is still unclear as to the effectiveness of creatine supplementation. However, it is important to mention that of 300 studies reviewed by Kreider, approximately 70% reported considerable beneficial effects from creatine supplementation. (20) With this majority of studies finding creatine supplementation increasing athletic performance, it is still important to investigate the varying types of creatine that are commercially sold. A couple of the more popular derivatives of creatine will be explored below.
Another factor that is to be considered with the different derivatives of creatine is the rate at which the creatine derivative breaks down into creatinine once it has entered the blood stream. Harris et al conducted a study on how three different types of creatine (creatine monohydrate, creatine citrate, and creatine pyruvate) affected creatine blood concentrations. (14) They found that the different types of creatine resulted in different blood creatine concentrations during a certain time period after supplementation. The results of their study are presented in Table 2:
Blood Creatine Concentration after Ingestion of Different forms of Creatine. Three male and three females were supplemented with the different forms of creatine using a capsule administration system. Each subject was given three different treatments, in which each treatment contained the different form of creatine. The amounts administered were 5g of creatine monohydrate, 6.7g creatine citrate, and 7.3g creatine pyruvate. The differing amounts were given to ensure each subject was given exactly 5g of creatine in each treatment. As one can see the different forms of creatine gave conflicting results. (14)
Because it has been shown that creatine monohydrate has approximately a 100% bioavailability, the researchers speculate these differences in blood concentrations is a result of varying rates of degradation of the different derivatives of creatine. (14)
The reason for these differing results lie within the molecular structure of each derivative of creatine. Each labeled structure is shown in Appendix 1. Creatine citrate is more soluble in water than creatine monohydrate, making it easier to administer a more consistent supplementation, when taken orally. Creatine citrate exists in the form of a salt, with a molecule of creatine ionically bonded with a citric acid molecule. This form of acidic creatine has a lower pKa of approximately 5 because of the citric acid molecule. This in turn raises the bioavailability slightly, as well as lowers the rate at which the creatine breaks down. This is a result of the acidic creatine being more stable in the acidic nature of the stomach. (15) Creatine pyruvate is very similar to the citric acid derivative in that it exists as a salt of creatine and pyruvic acid. (15) This modification again lowers the pKa of the creatine molecule making its bioavailability again slightly higher, heightened water solubility, and a slightly slower degradation rate into creatinine.
A new form of creatine that has become available in the last couple of years is the creatine ethyl ester (CEE). CEE exists as a creatine based compound where the carboxylic acid terminal is replaced by and ethyl ester. (2) Because creatine is degraded more rapidly in the acidic conditions of the stomach to creatinine than the ethyl ester derivative, the bioavailability of CEE is slightly higher than the monohydrate form. (2) This slower breakdown of CEE allows the body to utilize the full amount of creatine ingested. In addition, CEE has a higher lipophilicity causing it to have a much higher membrane permeability. (2) This allows for the CEE molecule to penetrate the muscle fibers with greater efficiency than the monohydrate derivative.
In 1927 phosphocreatine, a derivative of creatine, was discovered. This breakthrough discovery came from Fiske and Subbarow. (1) Their research established the fact that phosphocreatine concentrations in skeletal muscles decreased during contractions, and concentrations rose again during resting periods. (1) They also discovered the role phosphocreatine plays in energy production. After Fiske and Subbarow’s findings, little research was conducted on creatine until 1992 when Chanutin reported that upon creatine ingestion, total creatine concentrations in skeletal muscle rose. (1) This finding meant that supplemental creatine could be absorbed directly into skeletal muscle. This study has been confirmed by other studies including the one conducted by Harris et al in 1992, which is considered the major persuading study that began the revolution of creatine supplementation. (17) As a result of the Harris et al study, the amount of research being conducted on creatine increased rapidly. The purpose of this paper is to demonstrate how creatine can be used as a sports supplement using a chemical standpoint, and to investigate differing factors that contribute to the effectiveness of creatine supplementation.
In recent years, creatine has become an interesting topic outside of the performance enhancing field and into the medical field. Evidence has been revealed that suggests creatine may have importance in the treatment of several disorders such as Parkinson’s disease, neuromuscular diseases, brain diseases, muscular dystrophies, and even heart disease. (2,19) This evidence has broadened the possibility of creatine outside of improving athletic performance and into saving lives.
Creatine in the Human Body
As stated above, it has been shown that phosphocreatine concentrations fluctuate with muscle contraction. (1) This finding suggested the role that creatine plays in the human body. It has been speculated that creatine can ultimately raise that amount of energy humans “store” inside of their skeletal muscles. When a muscle contracts it uses the hydrolysis of a phosphoanhydride bond from an adenosine triphosphate (ATP) molecule to release energy. The hydrolysis of the ATP molecule yields an adenosine diphosphate (ADP) molecule. (3) This is the primary mechanism the human body uses to receive energy during muscle contraction. Because of this dependency on ATP hydrolysis, the concentration, and therefore the availability of ATP, in the skeletal muscle is critical in determining the amount of “stored” energy the muscle has to perform a contraction (in most cases exercise).It is important to note that when ATP is being dephosphorylated into ADP, resynthesis of ATP is being achieved at the same time inside of the muscle. This is where creatine becomes a crucial factor in the energy production of muscle cells in the body. During contraction, this resynthesis of ATP from ADP is accomplished through the degradation of phosphocreatine. This reaction is presented in figure 1: (4)
Figure 1:
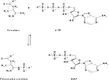
The Creatine Kinase Reaction Presented in this image is the creatine kinase reaction which occurs in skeletal muscle to resynthesize ATP. As one can see phosphocreatine combined with ADP yields creatine (Cr) and ATP to be used for energy production. Adapted from Wyss and Kaddurah-Daouk (2000). (4)
As a result of this direct interaction between phosphocreatine and ADP, phosphocreatine concentrations is the limiting factor in the resynthesis of ATP. With this knowledge of how skeletal muscle uses the creatine kinase reaction to produce energy, the supplementation of creatine can now be investigated. Many factors regarding creatine supplementation will be explored below, as well as the effectiveness of the creatine supplementation on performance enhancement.
The first factor that is to be investigated is the contribution creatine, in its pure form, has on maintaining energy levels. Even though creatine does not directly react with ADP to produce ATP, there is a pathway by which creatine assists in the resynthesis of ATP as shown in figure 1. Creatine performs this task by acting as a buffer to the reaction that occurs during the resynthesis of ATP. (5) It has been proven that upon creatine supplementation, the total concentration of phosphocreatine in skeletal muscle increases. (6) Therefore, creatine supplementation does not allow the concentration of phopsocreatine to decrease low enough where the equilibrium will shift away from the production of ATP under Le Chatelier’s principal. This elevated phosphocreatine also shifts the equilibrium towards production of ATP under Le Chatelier’s principal as well. This buffering system, in turn, allows for much faster and more efficient production of ATP, causing the overall of availability of ATP to rise.
Although one may wish to consider creatine as the perfect supplement for energy production in muscle tissue, reduced energy production is not the only reason muscle fibers become fatigued. The other factor to consider, with regard to muscle fatigue, is the build up of lactic acid (2-hydroxypropanoic acid) during contraction. As the muscle contracts the demand for ATP begins to rise. As ATP use increases, lactic acid is produced in the muscle tissue at a rate faster than the muscle can remove it. This inability to remove lactic acid fast enough causes the concentration of lactic acid in the muscle tissue to rise. As the concentration rises, this lowers the pH of the muscle with has been linked to cause a fatigue effect in the muscle. (7) However, it is important to note that according to Katz et al the fatigue that is experienced during short-term contraction is more dependant on low phosphocreatine concentrations rather than the increased concentrations of lactic acid. (8) This result has been confirmed by Saugen et al. (18) These findings show the importance phosphocreatine plays in the amount of energy a muscle can produce during contraction.
Another aspect of creatine in the human body to consider is which type of muscle fiber consumes more creatine during contraction. According to Casey and Greenhaff, the two different types of muscle fibers found in skeletal muscle, type I and type II, consume creatine in different amounts. (3) Type I fibers are considered slow-twitch fibers and are more useful when conducting aerobic exercise such as long distance running or biking. Type II fibers are considered fast-twitch fibers and are used during short maximum effort muscle contraction such as lifting weights. They report that the use of phosphocreatine in type II fibers can be as much as 33% higher than the use in type I fibers. Since the major source of energy for type I fibers is triglycerides and ATP for type II fibers, it can be speculated that the supplementation of creatine would be more beneficial for short explosive anaerobic exercises, than it would be for longer aerobic exercises. (3)
It is also important to investigate the maximum amount of creatine, and ultimately phosphocreatine, which can be stored inside muscle fibers. The average person who consumes a normal diet consisting of protein, carbohydrates, and fats has a base creatine level of approximately 120mmol of creatine per kilogram of dry mass. (5) This amount can be substantially lower depending on the individual’s diet. For example, a vegetarian has lower creatine levels because of the absence of meat in the individuals diet. According to Paddon-Jones et al, the maximum concentration of creatine in skeletal muscle that can be achieved through supplementation is 160mmol per kilogram of dry mass. (5) This concentration cannot be increased by additional creatine supplementation. Once this concentration has been reached, additional ingested creatine will be excreted.
In addition to the proposed energetic effects creatine has on skeletal muscle, it has been found to play a role in the mechanisms that control muscle protein synthesis. In one study, researchers discovered that elevated creatine concentration of skeletal muscle cells leads to an increase in myosin heavy chain synthesis. (9) These studies were conducted in vitro using tissue cultures. The results of Ingwall’s et al study are presented in Table 1.
Table 1:
<Experiment # | Total Protein synthesis (cmp/ g DNA) | Myosin heavy chain synthesis (cmp/ g DNA) | Percent increase in rate of myosin synthesis | |
Control | 1 | 149,000 | 360 | - |
2 | 134,000 | 350 | - | |
+5mM Creatine | 1 | 161,000 | 800 | 120 |
2 | 151,000 | 610 | 75 |
As one can see, the increase of creatine concentrations raised the amount of myosin heavy chain and the total protein synthesized inside of the cell. These findings were later confirmed by many similar studies conducted by Ingwall et al. (19) Because myosin heavy chain is the major protein that initiates muscle contraction, these results give evidence that creatine promotes maximum muscle contraction through two different mechanisms: an energetic effect as well as promoting the synthesis of myosin heavy chain. Conversely, it is also important to note that some studies have not been able to find that creatine supplementation improves overall protein synthesis. However, one such study was able to find that creatine supplementation possibly decreased the rate of protein catabolism, or the rate in which protein is broken down inside of the body. (19)
Even though the theoretical possibilities of creatine seem to be amazing, the question still remains as to whether creatine supplementation can improve athletic performance. There have been countless studies conducted on creatine supplementation, and surprisingly not, they have revealed conflicting results. Casey and Greenhaff found that creatine supplementation of 20g per day for five days resulted in improved performance in maximum isokinetic leg extensions of up to 4%. (3) Casey et al discovered that with the same supplementation regimen as Casey and Greenhaff, nine male subjects increased their total work production during several maximum isokinetic exercises by an average of 4%. (10) Harris et al conducted tests on mid-range trained runners at Tartu University, where they found creatine supplementation of 20-30g per day for five days significantly improved the runners’ times in their respective race compared to a placebo group. (11) This study conducted by Harris et al indicated that creatine was not only useful in short term maximum muscle contraction, but also useful in exercises that use more type I muscle fibers such as mid-ranged races.
Although there have been a large number of studies conducted where creatine supplementation was shown to improve athletic performance, there were many other studies where no such evidence was found. One such study was conducted by Syrotuik et al, in which they investigated the performance of rowers. (12) The study found that through an eight week period, there was no significant increase between the subjects supplementing with creatine and the placebo group. Similar results were obtained by Odland et al during 30 seconds of maximum exercise. (13) Because many of these studies tend to contradict each other, it is important to note some of the aspects of the studies presented above. The study conducted by Odland et al only lasted for three days, in which many feel is too short for the phosphocreatine concentrations inside the muscle to be properly elevated. The normal time period allowed for creatine concentration in the muscle to reach a maximum level and stay constant is five days of supplementation. This shortened time period could possibly lead to inaccurate results, and incorrect conclusions. The study performed by Syrotuik et al lasted significantly longer than the previous study. However, this study used the same training volume for both the supplemented group and the placebo group. As the supplemented group phosphocreatine concentrations began to rise, they were unable to increase their training volume if they wished throughout the study. Because the training volume was held constant for both groups in the study, this could have led to inaccurate results.
Through the results discussed above, it is still unclear as to the effectiveness of creatine supplementation. However, it is important to mention that of 300 studies reviewed by Kreider, approximately 70% reported considerable beneficial effects from creatine supplementation. (20) With this majority of studies finding creatine supplementation increasing athletic performance, it is still important to investigate the varying types of creatine that are commercially sold. A couple of the more popular derivatives of creatine will be explored below.
Derivatives of Creatine
Creatine monohydrate was the first form of creatine sold as a dietary supplement. (15) It exists with a creatine molecule hydrogen bound to a single water (hydrate) molecule. This is the simplest and most popular form of creatine sold. (15) Creatine monohydrate has also had a controversial history regarding its bioavailability and its effectiveness as a dietary supplement. Many believe that creatine monohydrate is not able to pass through the intestine with great efficiency, therefore having a low bioavailability. (4) For this reason, it is often recommended that creatine monohydrate be supplemented with simple carbohydrates to induce an insulin spike in which the creatine is thought to be absorbed with more efficiency. (3) Although this is a very common belief, Harris et al state that the bioavailability of creatine monohydrate is approximately 100%. (14) Another reason why creatine monohydrate is controversial is that creatine monohydrate has a low solubility in water at room temperature, approximately 14g per one liter of water. (15) This low solubility requires creatine to be mixed with a large amount of warm water to give a consistent uniform mixture. (15)Another factor that is to be considered with the different derivatives of creatine is the rate at which the creatine derivative breaks down into creatinine once it has entered the blood stream. Harris et al conducted a study on how three different types of creatine (creatine monohydrate, creatine citrate, and creatine pyruvate) affected creatine blood concentrations. (14) They found that the different types of creatine resulted in different blood creatine concentrations during a certain time period after supplementation. The results of their study are presented in Table 2:
Table 2:
Creatine Monohydrate | Creatine Citrate | Creatine Pyruvate | |
Time (h) | Mean Concentration (M) | Mean Concentration (M) | Mean Concentration (M) |
0.0 | 40.5 | 56.5 | 44.0 |
0.5 | 488.6 | 551.1 | 637.0 |
1.0 | 761.9 | 855.3 | 972.2 |
1.5 | 660.8 | 771.8 | 875.7 |
2.0 | 557.0 | 624.2 | 681.7 |
Because it has been shown that creatine monohydrate has approximately a 100% bioavailability, the researchers speculate these differences in blood concentrations is a result of varying rates of degradation of the different derivatives of creatine. (14)
The reason for these differing results lie within the molecular structure of each derivative of creatine. Each labeled structure is shown in Appendix 1. Creatine citrate is more soluble in water than creatine monohydrate, making it easier to administer a more consistent supplementation, when taken orally. Creatine citrate exists in the form of a salt, with a molecule of creatine ionically bonded with a citric acid molecule. This form of acidic creatine has a lower pKa of approximately 5 because of the citric acid molecule. This in turn raises the bioavailability slightly, as well as lowers the rate at which the creatine breaks down. This is a result of the acidic creatine being more stable in the acidic nature of the stomach. (15) Creatine pyruvate is very similar to the citric acid derivative in that it exists as a salt of creatine and pyruvic acid. (15) This modification again lowers the pKa of the creatine molecule making its bioavailability again slightly higher, heightened water solubility, and a slightly slower degradation rate into creatinine.
A new form of creatine that has become available in the last couple of years is the creatine ethyl ester (CEE). CEE exists as a creatine based compound where the carboxylic acid terminal is replaced by and ethyl ester. (2) Because creatine is degraded more rapidly in the acidic conditions of the stomach to creatinine than the ethyl ester derivative, the bioavailability of CEE is slightly higher than the monohydrate form. (2) This slower breakdown of CEE allows the body to utilize the full amount of creatine ingested. In addition, CEE has a higher lipophilicity causing it to have a much higher membrane permeability. (2) This allows for the CEE molecule to penetrate the muscle fibers with greater efficiency than the monohydrate derivative.